In today’s world, we’re surrounded by devices that rely on converting light into electrical signals. From fiber optic communication networks powering the internet to camera sensors in smartphones, photodiodes play a crucial role in making this conversion possible.
Photodiodes are optoelectronic devices that transform light energy into electrical current. They’re tiny switches that turn on when light hits them. Let’s explore the different types of photodiodes and how they work.
Table of Contents
Types of Photodiodes
There are various types of photodiodes, each with its own advantages and disadvantages. Here are three common types:
- P-N Photodiode: The simplest type of photodiode. They are relatively inexpensive but have limitations, such as lower efficiency and slower response time.
- P-I-N Photodiode: An improvement over the P-N photodiode. They have a wider depletion region, leading to higher efficiency and faster response time. This makes them better suited for high-speed applications like fiber optic communication.
- Avalanche Photodiode: The avalanche photodiode (APD) takes the concept of a photodiode a step further. It utilizes an internal gain mechanism to significantly amplify the electrical current generated by incoming light. This makes APDs incredibly sensitive light detectors, ideal for applications where weak light signals need to be detected.
- Schottky photodiodes: Schottky photodiodes offer a distinct approach to light detection with advantages like fast response and potential for specific wavelength sensitivity. However, their efficiency for certain light spectrums can be lower compared to P-N or P-I-N photodiodes.
- MSM photodetectors: MSM photodetectors, also known as Metal-Semiconductor-Metal (MSM) photodetectors, are another type of device used to convert light into electrical current. They differ from the photodiodes (P-N, P-I-N, and Schottky) in their structure and operating principle.
P-N Photodiode
The P-N photodiode is the foundation for all other photodiode designs. It’s a relatively simple device made from a semiconductor material like silicon.
A P-N photodiode has two main regions: a P-region with positive charge carriers (holes) and an N-region with negative charge carriers (electrons). These regions meet at the P-N junction.
To operate, the P-N junction needs reverse bias. This means connecting the positive battery terminal to the N-region and the negative terminal to the P-region. This creates a depletion region around the junction with few mobile charge carriers.
Here’s how P-N Photodiode works:
- Light Absorption: Light shines on the photodiode, and some get absorbed within the depletion region.
- Electron-Hole Pair Creation: The absorbed light energy excites electrons, lifting them to higher energy levels. This creates an “electron hole” where the electron used to be.
- Current Generation: The electric field pulls them apart if an electron-hole pair is created within the depletion region. The freed electron moves towards the N-region, and the hole drifts towards the P-region. This charge movement constitutes an electrical current flowing in the external circuit.
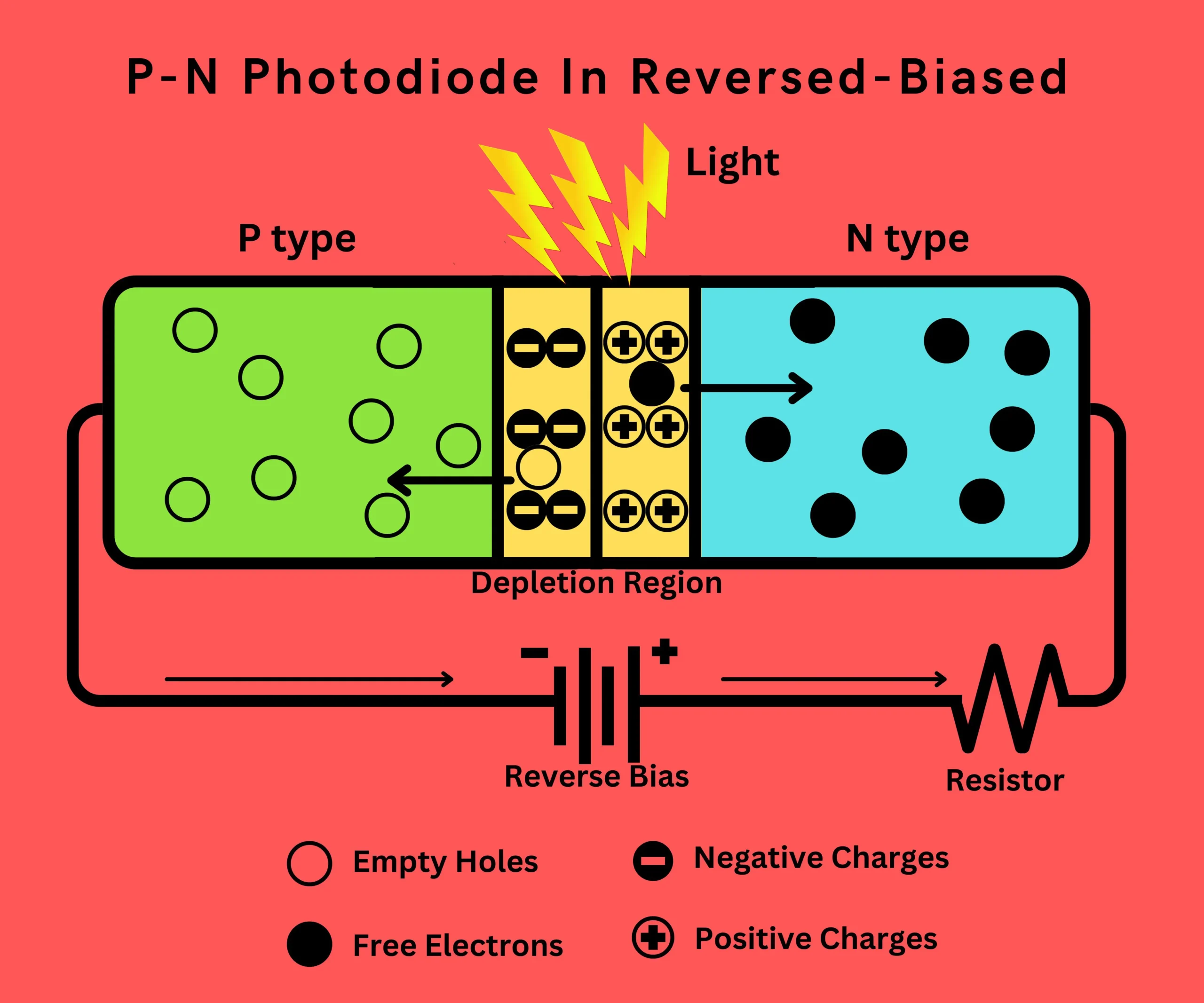
While P-N photodiodes are simple and inexpensive, they have limitations:
- Lower Efficiency: Not all absorbed light creates electron-hole pairs within the depletion region, leading to lower overall efficiency.
- Slower Response Time: The movement of electrons and holes takes time, limiting the speed at which a P-N photodiode can respond to changes in light intensity.
Facts about P-N Photodiodes
- The simplest type of photodiode
- Made from semiconductor materials like silicon
- Two main regions: P-region (holes) and N-region (electrons)
- Requires reverse bias for operation
- Creates a depletion region around the P-N junction
- Absorbed light creates electron-hole pairs within the depletion region
- Electron-hole pair separation generates electrical current
- Lower efficiency and slower response time compared to other photodiode types
P-I-N Photodiode
The P-I-N photodiode builds upon the basic concept of the P-N photodiode but overcomes some limitations. It’s a highly efficient light detector used in various applications like fiber optic communication.
A P-I-N photodiode has three main regions:
- P-region: Similar to a P-N photodiode, it has positive charge carriers (holes).
- Intrinsic (I) region: This middle layer is made from a lightly doped semiconductor material. It has few mobile charge carriers, creating a wider depletion region than a P-N diode.
- N-region: Similar to a P-N photodiode, it has negative charge carriers (electrons).
The wider depletion region in a P-I-N photodiode increases the chance that light-generated electron-hole pairs are within the influence of the electric field. This electric field separates the pairs, and the freed electrons and holes are swept toward their respective regions, creating a stronger electrical current compared to a P-N photodiode.
Key Advantages of P-I-N Photodiodes
- Higher Efficiency: The wider depletion region allows for more efficient absorption of light and conversion into electrical current.
- Faster Response Time: The shorter distance electrons and holes need to travel within the depletion region leads to a faster response to changes in light intensity. This makes P-I-N photodiodes suitable for high-speed applications.
Applications of P-I-N Photodiodes
P-I-N photodiodes are widely used in:
- Fiber Optic Communication: Receive weak light signals transmitted through optical fibers and convert them efficiently into electrical signals for processing information.
- High-Speed Detectors: Applications requiring fast response, like barcode scanners or optical receivers in data transmission systems.
- Medical Imaging: Some medical imaging techniques, like positron emission tomography (PET) scans, use P-I-N photodiodes to detect faint light signals.
Structure and Working Principle of P-I-N Photodiodes
Here’s a breakdown of how a P-I-N photodiode works:
- Reverse Bias: Just like the P-N photodiode, a P-I-N photodiode needs to be in reverse bias for proper operation.
- Light Absorption: Light shines on the photodiode, and some of it gets absorbed within the depletion region, primarily due to the wider intrinsic layer.
- Electron-Hole Pair Creation: Similar to the P-N photodiode, absorbed light energy excites electrons, creating electron-hole pairs.
- Efficient Current Generation: The wider depletion region increases the chance that light-generated electron-hole pairs are within the influence of the electric field. This electric field separates the pairs, and the freed electrons and holes are swept toward their respective regions, creating a stronger electrical current.
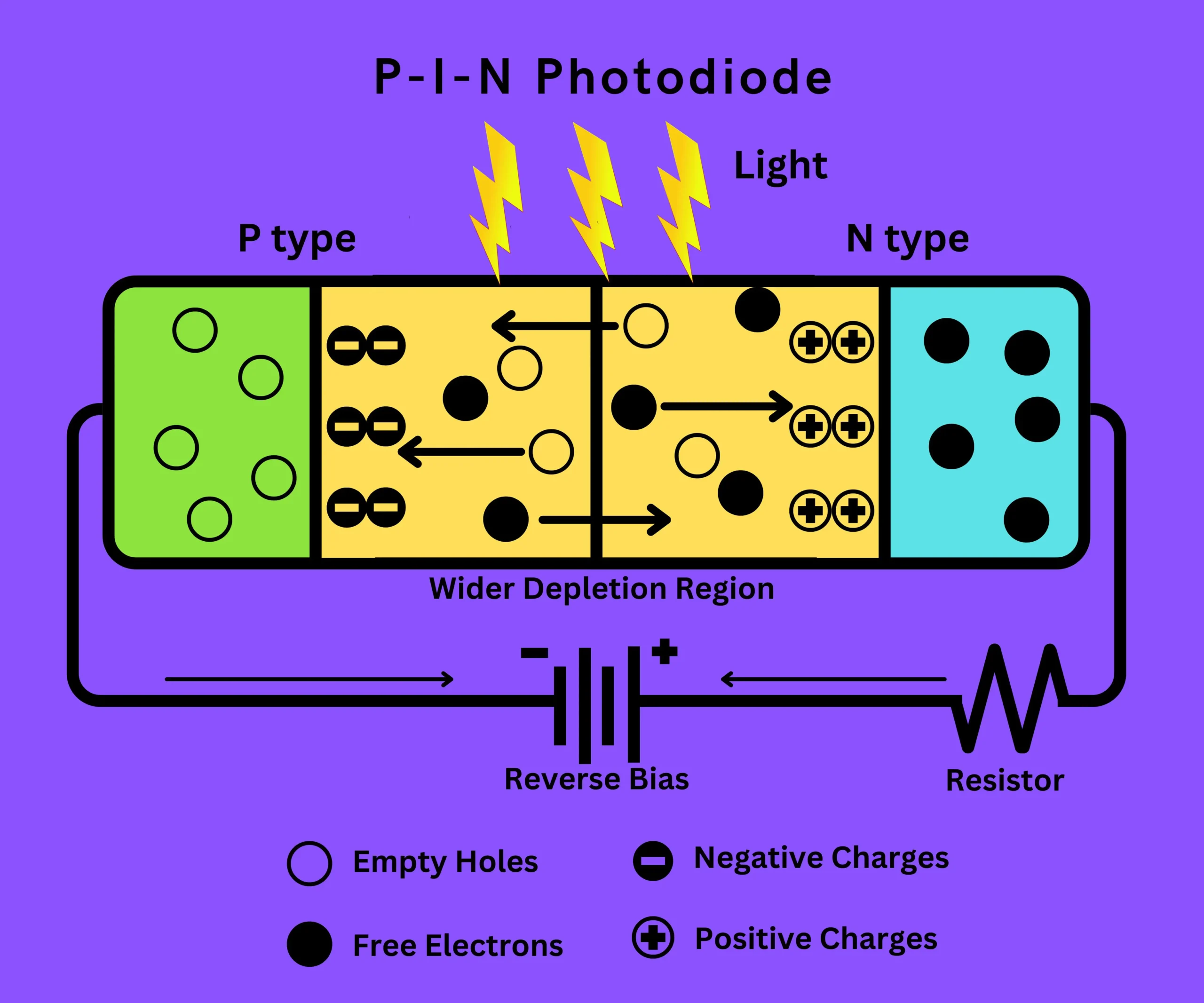
Avalanche Photodiode
The avalanche photodiode (APD) takes the concept of a photodiode a step further. It utilizes an internal gain mechanism to significantly amplify the electrical current generated by incoming light. This makes APDs incredibly sensitive light detectors, ideal for applications where weak light signals need to be detected.
An APD resembles a P-I-N photodiode but with a crucial difference. It has a specially designed high-field region within the depletion region.
How an Avalanche Photodiode Works
- Reverse Bias: Similar to other photodiodes, an APD needs to be in reverse bias for operation.
- Light Absorption: Light shines on the photodiode, and some of it gets absorbed within the depletion region, just like in a P-I-N photodiode.
- Electron-Hole Pair Creation: Absorbed light energy excites electrons, creating electron-hole pairs.
- Internal Gain through Avalanche Multiplication: This is the magic of APDs. When an electron-hole pair is created within the high-field region, the strong electric field accelerates these charged particles. These high-speed electrons can collide with neutral atoms in the material, creating new electron-hole pairs. This chain reaction of electron multiplication is called avalanche multiplication.
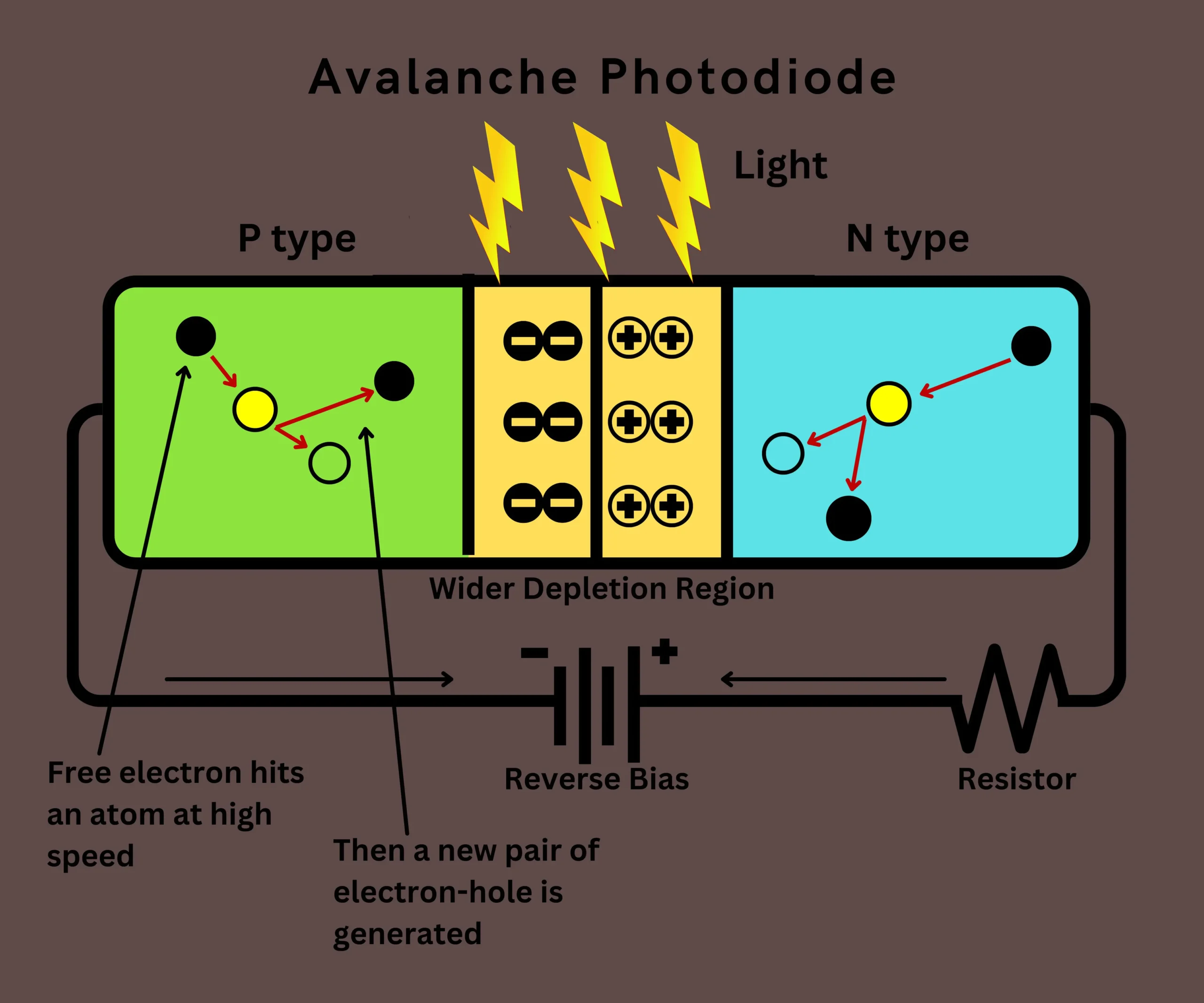
Key Points about Avalanche Multiplication
- The multiplication factor of an APD determines how much the current is amplified. This factor can range from tens to hundreds, significantly boosting the signal compared to a regular photodiode.
- The multiplication factor is not constant. It depends on the applied reverse bias voltage. Higher voltage leads to a higher electric field and a greater chance of avalanche multiplication but also increases the risk of unwanted electrical breakdown.
Applications of Avalanche Photodiodes
APDs are valuable in applications where weak light signals need to be detected, such as:
- Fiber Optic Communication: APDs can amplify faint received signals for better data transmission, especially for long-distance communication where light signals become weaker.
- Optical Receivers: APDs can improve signal reception by amplifying weak optical signals in various data transmission systems, like high-speed data links.
- Night Vision Devices: APDs can amplify faint starlight or other low-light sources, enhancing night vision capability.
- Medical Imaging Techniques: Some medical imaging applications, like certain types of microscopy, benefit from the high sensitivity of APDs to detect weak light signals.
Important Considerations for APDs
- Trade-off between Gain and Noise: While APDs offer high gain, avalanche multiplication can also introduce some electrical noise. This needs to be considered depending on the specific application.
- Breakdown Voltage: Operating an APD too close to its breakdown voltage can lead to unwanted current even without light present. Finding the optimal operating voltage is crucial.
Avalanche photodiodes provide exceptional sensitivity for detecting weak light signals. However, understanding the trade-offs between gain, noise, and operating voltage is essential for using them effectively.
Schottky Photodiodes
Schottky photodiodes are another type of photodetector, but unlike the ones we previously discussed, they rely on a different principle to convert light into electricity. Let’s break it down:
Working Principle Schottky Photodiodes
A Schottky photodiode utilizes a metal-semiconductor junction instead of a p-n junction. When light shines on the device, some of it gets absorbed in the semiconductor region.
If the energy of the absorbed light is high enough, it can excite electrons from the semiconductor, allowing them to overcome the potential barrier formed at the metal-semiconductor junction and enter the metal. This flow of electrons across the junction constitutes an electrical current.
Key Differences from P-N Junctions
- No Depletion Region: Unlike P-N junctions, Schottky diodes don’t have a depletion region. This can be an advantage as it allows for faster response times.
- Two Modes of Light Detection: Schottky photodiodes can operate in two ways – through electron-hole pair generation (similar to P-N junctions) and through the emission of electrons from the metal into the semiconductor due to absorbed light (called internal photoemission).
Applications Schottky Photodiodes
Schottky photodiodes are often used for:
- Ultraviolet (UV) and Visible Light Detection: The metal layer can be chosen to be sensitive to specific wavelengths, making it suitable for UV or blue light detection.
- High-Speed Applications: The lack of a depletion region allows for faster response times compared to P-N junctions.
Limitations of Schottky Photodiodes
- Lower Efficiency at Longer Wavelengths: The metal layer can reflect some light, especially at longer wavelengths like infrared, reducing overall efficiency.
Overall, Schottky photodiodes offer a distinct approach to light detection with advantages like fast response and potential for specific wavelength sensitivity. However, their efficiency for certain light spectrums can be lower compared to P-N or P-I-N photodiodes.
Relevance to Fiber Optic Communication
While Schottky photodiodes have some advantages like fast response time, they are generally not considered the most relevant choice for fiber optic communication. Here’s why:
- Lower Responsivity: Compared to P-I-N photodiodes, which are the workhorse for fiber optic communication, Schottky photodiodes typically have lower responsivity. Responsivity refers to the amount of electrical current generated per unit of light received. Maximizing responsivity is crucial in fiber optics, where signal strength can be weak after traveling long distances.
- Material Limitations: The metal layer in a Schottky photodiode can be sensitive to certain wavelengths, making it more suitable for ultraviolet (UV) or visible light detection. Fiber optic communication primarily uses infrared light wavelengths, for which Schottky photodiodes may not be as efficient.
- Focus on High-Speed Applications: While the lack of a depletion region offers faster response times in Schottky photodiodes, high speed isn’t always the top priority in fiber optic communication. Signal integrity and maintaining a strong signal are often more critical. P-I-N photodiodes can achieve sufficient speed for fiber optic applications while offering better responsivity for infrared light.
Therefore, while Schottky photodiodes have their own niche applications, P-I-N photodiodes remain the preferred choice for their overall efficiency and suitability for the specific wavelengths used in fiber optic communication.
MSM Photodetectors
MSM photodetectors, also known as Metal-Semiconductor-Metal photodetectors, are another type of device used to convert light into electrical current. Their structure and operating principle differ from the photodiodes we’ve discussed earlier (P-N, P-I-N, and Schottky).
Structure
MSM photodetectors consist of a layer of semiconductor material sandwiched between two interdigitated metal electrodes. These electrodes have their fingers closely spaced and facing each other but not actually touching.
Working Principle of MSM Photodetectors
- Light shines on the device, and it gets absorbed within the semiconductor region.
- If the absorbed light has enough energy, it excites electrons in the semiconductor, creating electron-hole pairs.
- The applied bias voltage across the electrodes creates an electric field within the semiconductor.
- These electron-hole pairs, under the influence of the electric field, separate and drift toward their respective electrodes (electrons to the positive electrode and holes to the negative electrode).
- This movement of charges constitutes an electrical current flowing in the external circuit.
Key Characteristics
- Fast Response Time: Due to the absence of a depletion region (unlike P-N junctions), MSM photodetectors can offer very fast response times. This makes them suitable for high-speed applications.
- Simple Fabrication: The interdigitated metal structure can be relatively simple to fabricate compared to some other photodetectors.
Limitations
- Lower Responsivity: Compared to P-I-N photodiodes, MSM photodetectors generally have lower responsivity. This means they generate a weaker electrical current for a given amount of light received.
- Higher Dark Current: Even without light present, there can be some leakage current in MSM photodetectors due to various mechanisms. This can affect the signal-to-noise ratio.
Applications of MSM Photodetectors
MSM photodetectors are sometimes used in:
- High-Speed Optical Communication Systems: Their fast response time can be beneficial in specific scenarios, although P-I-N photodiodes are still more common.
- Microwave Detection: They can be used to detect high-frequency electromagnetic waves in the microwave range.
- Imaging Applications: In some specialized imaging applications, their fast response and simple fabrication may be advantageous.
MSM Photodetectors in Fiber Optic Communication
While MSM photodetectors have some relevance to fiber optics, P-I-N photodiodes are the dominant choice for several reasons:
- Lower Responsivity: A crucial factor in fiber optics is maximizing the conversion of weak light signals into a strong electrical current. MSM photodetectors generally have lower responsivity compared to P-I-N diodes, making them less efficient for capturing faint light signals that travel long distances in fiber optic cables.
- Higher Dark Current: MSM photodetectors can experience leakage current even in darkness, adding noise to the signal, which is undesirable in sensitive fiber optic communication systems.
- Focus on Different Wavelengths: While MSM photodetectors can be tailored for various wavelengths, fiber optic communication primarily uses infrared wavelengths. P-I-N photodiodes are generally optimized for these wavelengths and offer better performance.
Overall, while MSM photodetectors have a niche in high-speed applications, P-I-N photodiodes remain the preferred choice for fiber optic communication due to their superior responsivity, lower dark current, and better match to the infrared wavelengths used in fiber optics.
Here’s a comparison table for the different types of photodetectors we’ve discussed
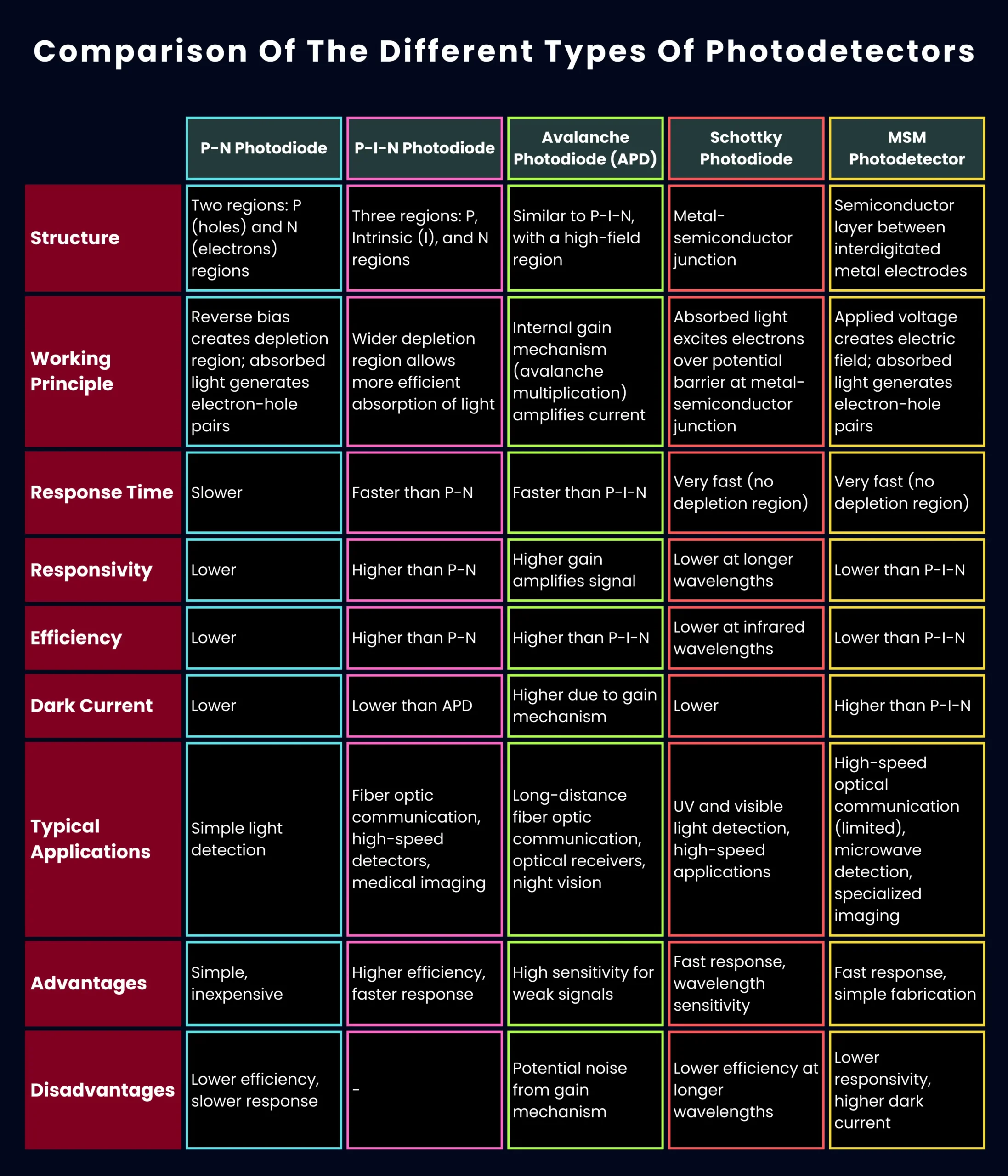
Conclusion
Photodetectors play a crucial role in converting light energy into electrical signals that power numerous technologies around us. The different types of photodetectors – P-N, P-I-N, avalanche, Schottky, and MSM – each offer unique strengths and find applications in diverse fields.
For fiber optic communication systems that transmit data over long distances, the P-I-N photodiode emerges as the preferred choice. Its wide depletion region allows efficient light absorption and conversion into strong electrical currents, even for faint incoming signals. Additionally, P-I-N photodiodes achieve high speeds that are suitable for data transmission while maintaining superior responsivity.
Avalanche photodiodes provide exceptional sensitivity by internally amplifying weak light signals through avalanche multiplication. This exceptional gain makes them valuable for long-haul fiber optic links and applications like night vision, where detecting faint light is paramount.
While Schottky photodiodes offer fast response times and wavelength selectivity, their lower efficiency at infrared wavelengths limits their usage in fiber optics. Similarly, MSM photodetectors, though fast, suffer from lower responsivity and higher noise, restricting their fiber optic applications.
FAQ
Which photodiodes are used as fiber optic detectors?
The primary photodiodes used in fiber optic detectors are P-I-N photodiodes. These offer a good balance of high responsivity (converting light efficiently into electrical current) and fast response time. This is crucial for capturing weak light signals that travel long distances in fiber optic cables.
What are the most commonly used photodetectors?
P-I-N photodiodes are some of the most commonly used photodetectors, especially in fiber optic communication due to their high responsivity and suitability for infrared wavelengths.
Do photodiodes use the photoelectric effect?
Yes, photodiodes rely on the photoelectric effect. This principle states that light can eject electrons from certain materials. In a photodiode, the absorbed light energy excites electrons, allowing them to be separated by an electric field and generate an electrical current.
Can photodiodes detect visible light?
Yes, many photodiodes, including P-I-N photodiodes, can detect visible light. However, the sensitivity may vary depending on the specific material used in the photodiode and the color of the light. Fiber optic communication primarily uses infrared light wavelengths, for which P-I-N photodiodes are well-optimized.
Is msm photodetector and photodiode or semiconductor?
An MSM photodetector is both a photodiode and a semiconductor device. It utilizes the properties of a semiconductor material to create a photodiode functionality. It leverages the interaction of light with the semiconductor to create an electrical current.
Audemars Piguet’s Royal Oak 15450ST boasts a
slim 9.8mm profile and 5 ATM water resistance, blending sporty durability
Its silvery-grey Grande Tapisserie dial includes luminescent hour markers and a scratch-resistant sapphire crystal, ensuring legibility and resilience.
Powered by the caliber 3120 movement, it offers a reliable 60-hour reserve for uninterrupted precision.
Introduced in the early 2010s, the 15450ST complements the larger 41mm 15400 model, catering to classic proportions.
The recent 2019 iteration highlights enhanced detailing, appealing to collectors.
https://biiut.com/read-blog/1784
A sleek silver index dial with Grande Tapisserie highlighted by luminous appliqués for effortless legibility.
The stainless steel bracelet offers a secure, ergonomic fit, finished with an AP folding clasp.
Renowned for its iconic design, it continues to captivate collectors among luxury watch enthusiasts.