Fiber optic communication relies on laser diodes as optical sources to create light signals that carry information through cables. Laser diodes can be made from semiconductor materials that emit light when a voltage is applied across their p-n junction. They produce a stimulated emission process that results in the amplification of light. The term “laser” comes from the acronym for “light amplification by stimulated emission of radiation.”
In this article, let’s examine the structure and operation of laser diodes. Also, categories of main laser diode types that are used in fiber optics by their mode of operation and design structure. Additionally, let’s discuss technical details on single-mode, multi-mode, edge-emitting, surface-emitting, quantum well, and other laser diode varieties.
Table of Contents
‘LASER’ Meaning
The term laser is an acronym for “light amplification by stimulated emission of radiation.” This phrase summarizes the unique light generation process occurring in lasers. So, let’s look at the meaning word by word from the descending order.
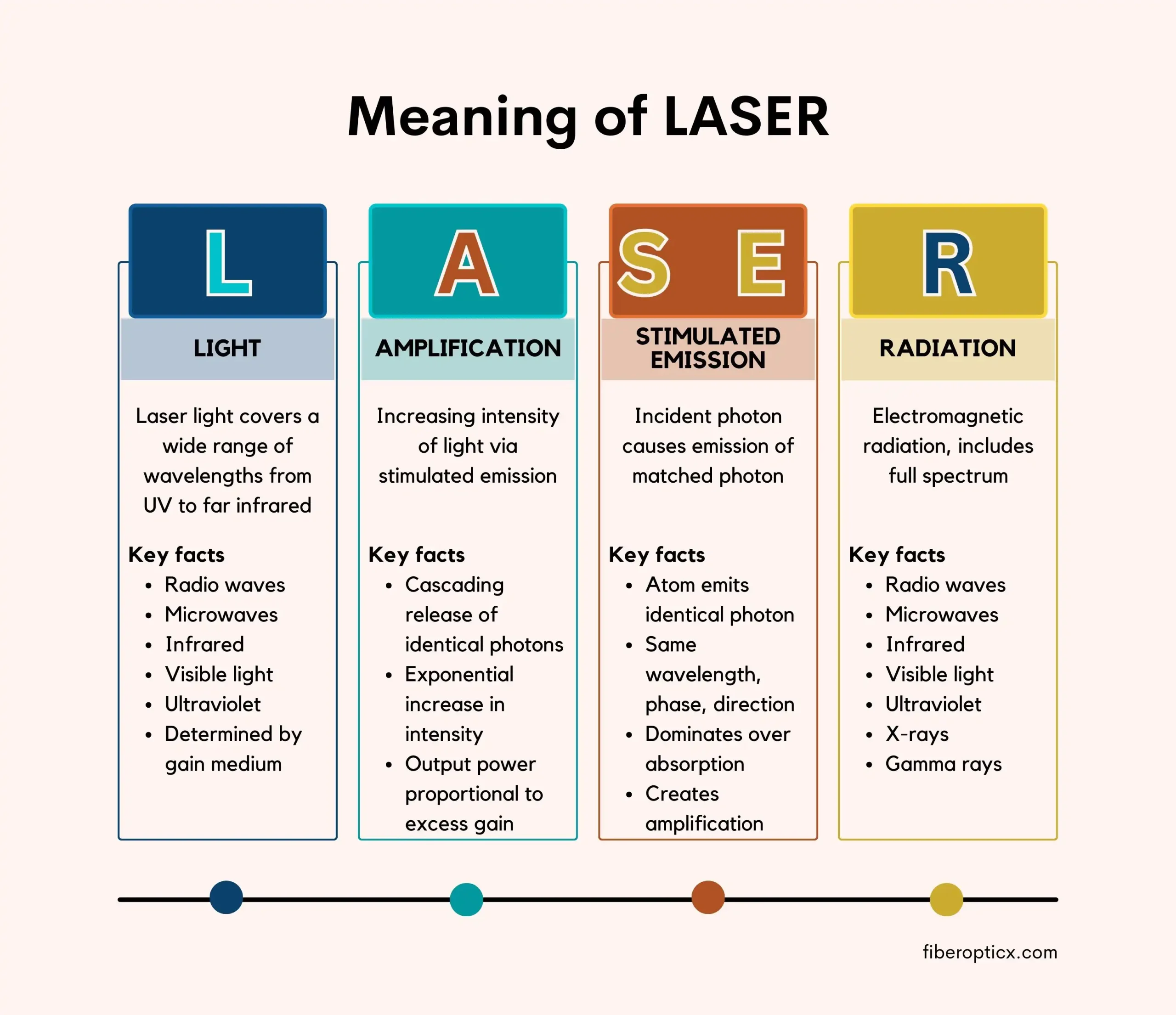
Radiation
Radiation refers to electromagnetic radiation, which is a form of energy that can propagate as waves or discrete particles called photons. It moves at the speed of light. Laser radiation includes visible light but also embraces other electromagnetic waves like infrared, ultraviolet, X-rays, and microwaves.
- Laser radiation has properties of both waves and particles.
- It can be described in terms of wavelength, frequency, amplitude, and speed.
- As particles, laser light consists of photons that carry discrete amounts of energy.
- Radiation from coherent laser sources exhibits higher directionality and intensity than ordinary incoherent light.
- Focusing laser radiation into a narrow beam enables many of its useful applications.
- The radiation produced by a laser depends on the gain medium used and can range from ultraviolet to far infrared wavelengths.
- Laser radiation is usually near-monochromatic, meaning it contains just a single wavelength or a very narrow band of wavelengths.
So, the term radiation in LASER refers to the coherent, directed electromagnetic waves or beams of photons emitted through stimulated emission.
Laser radiation can span the electromagnetic spectrum from radio waves to X-rays, depending on the gain medium. Its high intensity and narrow spectral purity compared to ordinary light make laser radiation useful for fiber optic communications.
Stimulated emission
Stimulated emission is the key physical principle that enables light amplification in lasers. It differs from spontaneous emission, where light is released randomly from atoms or molecules.
- In stimulated emission, an incoming photon causes an atom/molecule to release an identical photon.
- The initial light wave stimulates the emission of another matched light wave.
- The emitted light has the same wavelength, phase, polarization, and direction as the incident-stimulating photon.
- Stimulated emission amplifies the light signal as more photons are released.
- In lasers, stimulated emission dominates over absorption processes due to population inversion.
- This allows laser light to reflect back and forth in the cavity, being amplified each pass.
So stimulated emission is vital to producing the directed, monochromatic light beam emitted by lasers through the cascading release of identical photons. It enables the light amplification process central to laser operation.
Amplification
Amplification refers to the increase in light intensity that occurs in a laser.
- It results from stimulated emission releasing multiple identical photons.
- Each emitted photon can stimulate another atom to release a matched photon.
- This cascading effect leads to exponential light amplification as it reflects in the laser cavity.
- Amplification continues until the laser reaches a threshold where the gain equals the losses.
- Laser output is proportional to the excess gain above the threshold.
- High amplification gives laser light much greater intensity than ordinary incoherent light sources.
So amplification through stimulated emission is the key process allowing lasers to generate an intense, directed beam from a weak initial signal. The cascading release of identical photons makes the light output much brighter.
Light
Light refers to the type of electromagnetic radiation produced by lasers, which spans near-ultraviolet to far-infrared wavelengths.
- Lasers emit light in the visible range but also at shorter wavelengths like ultraviolet or longer wavelengths such as infrared.
- The gain medium determines the wavelength of laser light during the emission process.
- Semiconductor laser diodes typically emit infrared light around 1300 nm or 1550 nm, which are useful wavelengths for fiber optics.
- Other lasers based on different gain media can produce ultraviolet, visible, or mid-infrared light.
- Laser light is characterized by high directionality, brightness, and spectral purity compared to ordinary incoherent light.
The term ‘light’ encompasses the wide range of wavelengths in the electromagnetic spectrum that can be produced through stimulated emission and amplification in different laser gain media. The light also exhibits exceptional coherence and intensity compared to normal light sources.
Basic Structure and Operation of Laser Diodes
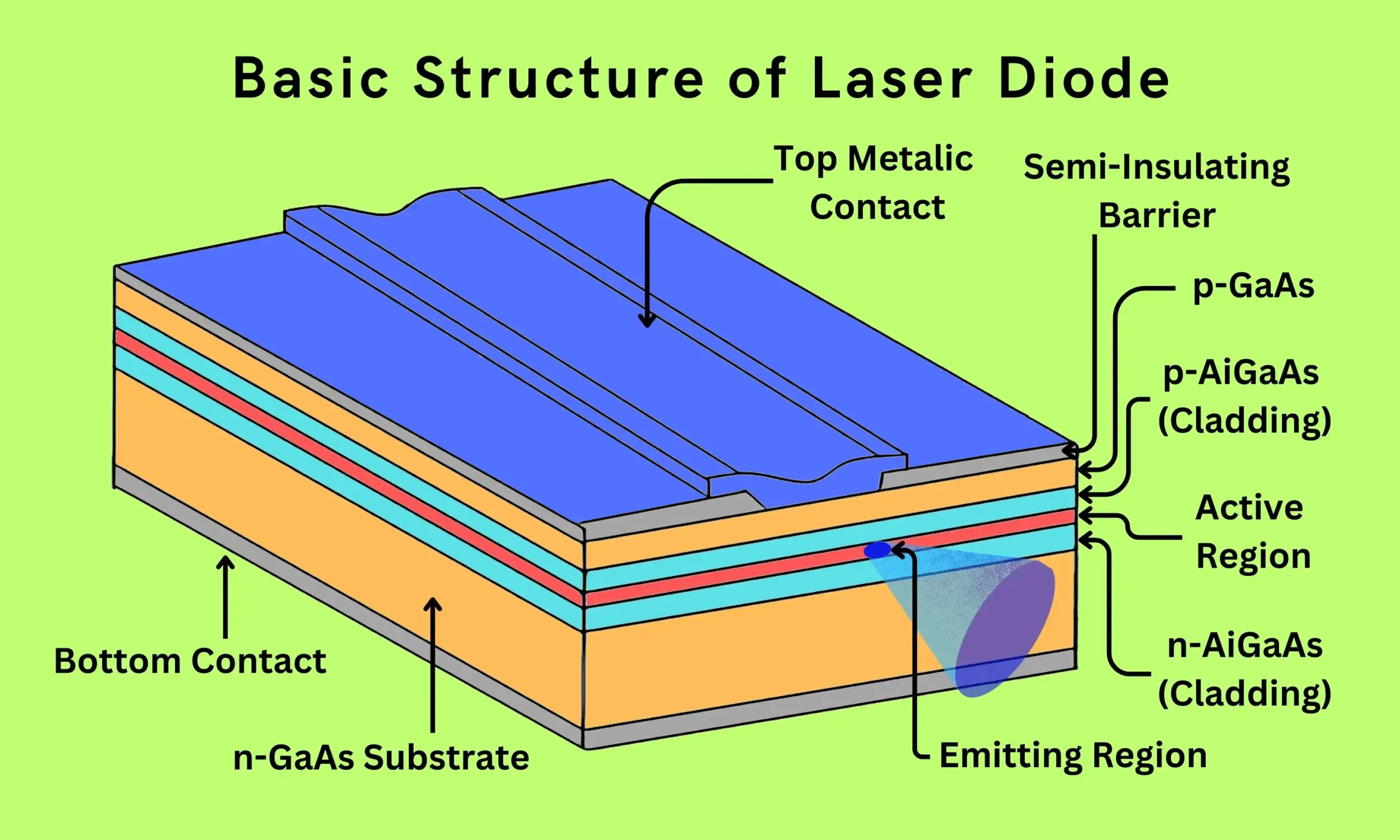
Laser diodes contain some key components and operate through a unique light generation process.
- The active layer where light is emitted is sandwiched between two cladding layers of opposite doping types.
- Applying forward voltage across the p-n junction causes electrons and holes to recombine in the active layer, generating photons through spontaneous emission.
- The lower refractive index of the cladding layers confines the emitted light within the higher-index active layer through total internal reflection.
- The edges or facets of the active layer act as mirrors, forming an optical cavity for the laser.
- Light reflects back and forth between the mirrors, being amplified by stimulated emission.
- At threshold current, the round trip gain equals losses, and laser oscillation occurs.
- Further increasing current increases the power of coherent laser light emitted from one facet.
Laser diodes consist of an active light-emitting layer surrounded by lower-index cladding layers within a reflective optical cavity. Spontaneous emission initiates the laser process, while stimulated emission provides amplification to reach the lasing threshold and coherent laser output.
Classification of Laser Diodes
Laser diodes can be categorized in different ways based on their properties and structure. Two main classification methods are:
By Mode of Operation:
- Single-mode laser diodes: Emit a narrow beam on one frequency/spatial mode.
- Multi-mode laser diodes: Emit a wider beam with multiple frequencies/modes.
By Structure/Design:
- Edge-emitting laser diodes: Active layer and emission parallel to the wafer surface.
- Vertical-cavity surface-emitting laser diodes: Emission perpendicular to active layer surface.
These categories encompass the wide range of laser diode types and configurations used for different applications like optical fiber communications.
The specifics of each laser diode variety are determined by factors like the semiconductor materials used, the presence of wavelength-selecting elements, crystal orientation, and beam-shaping optics. Their classification provides a framework to understand the nuances of diode laser design.
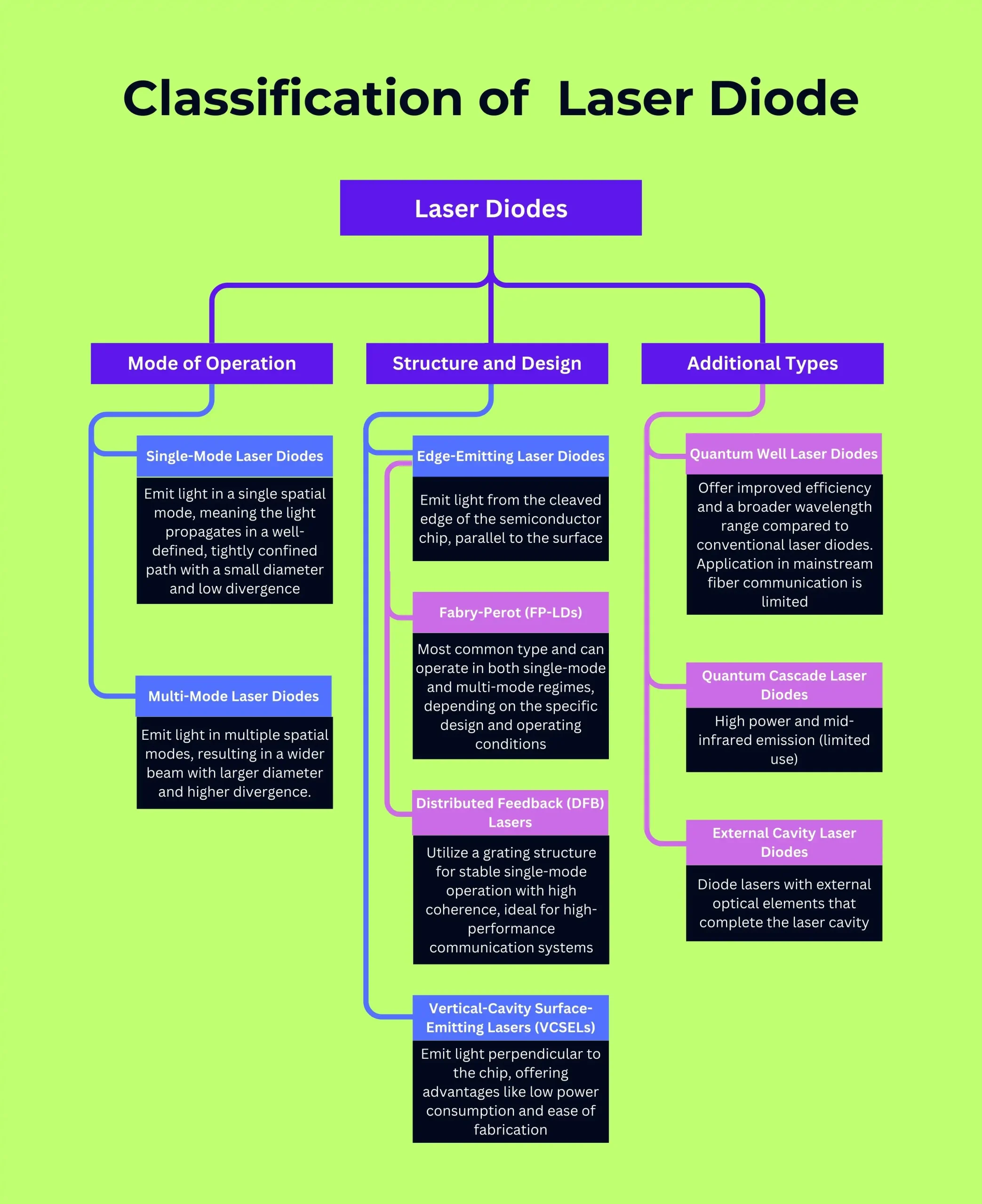
Laser Diode Types by Mode of Operation
Laser diodes can be classified based on their mode of operation as either single-mode or multi-mode. This refers to the number of wavelengths and spatial patterns produced in the emitted laser beam.
Single-Mode Laser Diodes
- Emit light on just one dominant frequency and spatial mode.
- Produce a narrow, intense beam profile.
- Exhibit very high coherence with all light waves in phase.
- Have a narrow linewidth or wavelength spread under 0.01 nm.
- Low beam divergence allows coupling into single-mode fibers.
- Mainly used for fiber-optic communications over long distances.
So, single-mode laser diodes generate a tightly focused beam on a single optical frequency. Their high coherence makes them ideal for long-haul fiber optic telecom networks.
Multi-Mode Laser Diodes
- Emit multiple wavelengths simultaneously in many spatial modes.
- Produce a wider beam profile with lower intensity.
- Light waves have random phase differences.
- Large linewidths over 2-10 nm.
- Higher divergence means more difficult fiber coupling.
- Used for short-reach networks and industrial applications.
In contrast, multi-mode laser diodes emit broader beams with multiple wavelengths and modes. Their lower coherence is acceptable for shorter-distance data transmission in centers and campuses.
So, single-mode lasers provide long-distance capability, while multi-mode lasers are preferable for short-reach uses. Matching the diode laser type to the network requirements is an important consideration for fiber optic systems.
Laser Diode Types by Structure/Design
Laser diodes fall into categories of edge-emitting and surface-emitting based on their structural orientation. Within these groups are specific varieties like Fabry-Perot and VCSELs.
Edge-Emitting Diode Lasers
The active region in edge-emitting lasers is a thin layer of light-emitting semiconductor material like gallium arsenide sandwiched between two larger cladding layers of a lower refractive index material.
When current is injected across the junction, photons are emitted in the active layer and reflected back and forth between the cleaved facet mirrors.
Edge emitters utilize a waveguide structure to confine the optical mode closely to the gain region and control the beam shape. The refractive index distribution determines the transverse mode structure.
The ends of the active layer are cleaved to form smooth, parallel mirrors. The reflectivity of the mirrors is typically 30-50%.
Edge emitters can achieve very high gain in a small volume but require precise cleaving and packaging. The emitting area is large in one dimension, producing an elliptical beam profile.
Fabry-Perot Laser Diodes
Fabry-Perot lasers have an optical cavity defined by the cleaved facets acting as partially reflecting mirrors. The facet reflectivity and cavity length determine the lasing wavelengths. Mode spacing is very wide, ~1 nm.
Optical feedback is purely by facet reflection. No wavelength-selective elements are included. It is prone to mode hopping since many axial modes exist within the gain spectrum. Noise results from switching between modes.
Typical output power of 10-20 mW. Mainly used for short, low-speed optical links. Low cost compared to DFB lasers.
Distributed Feedback Laser Diodes
DFB lasers include a diffraction grating fabricated in the active region, which acts as a wavelength-selective element. The grating provides optical feedback, causing a single longitudinal mode to oscillate. Side modes are suppressed.
The Bragg wavelength is governed by the grating period. Wavelengths meeting the Bragg condition see net gain while others experience loss. DFB lasers maintain single-frequency operation since the grating fixes the lasing wavelength.
Typical linewidth under 5 MHz. Output power up to 100 mW. Used for long-haul telecom.
Vertical-Cavity Surface-Emitting Laser
VCSELs use ultra-high reflectivity dielectric mirror stacks above and below the thin active region to form the laser cavity perpendicular to the wafer surface. Its cavity length is short, usually ~1 wavelength, enabling high longitudinal mode spacing for single-frequency operation.
VCSEL’s optical confinement occurs through index guiding, with the oxide layers providing refractive index steps. Its emission area is circular, producing a symmetric Gaussian beam profile for efficient coupling into fibers.
Advantages of VCSEL diodes include low divergence, high beam quality, wavelength stability, and inexpensive high-volume manufacture.
VCSELs are widely used in short-reach optical interconnects like data centers. Powers from 1-10 mW.
Limitations of Surface-Emitting Diode Lasers
While vertical-cavity surface-emitting lasers (VCSELs) are widely used, basic surface-emitting diode lasers (SELDs) have limitations, including lower gain and difficulty producing high-quality beams from large emitting areas. So, SELDs have not replaced dominant edge-emitting diode lasers for long-haul fiber optic networks.
Redundancy of Distributed Bragg Reflection Lasers
Distributed Bragg reflection (DBR) lasers are functionally similar to distributed feedback (DFB) lasers but are less efficient due to the inactive grating region. DFB lasers provide effective wavelength control for single-mode fiber networks, making DBR lasers redundant.
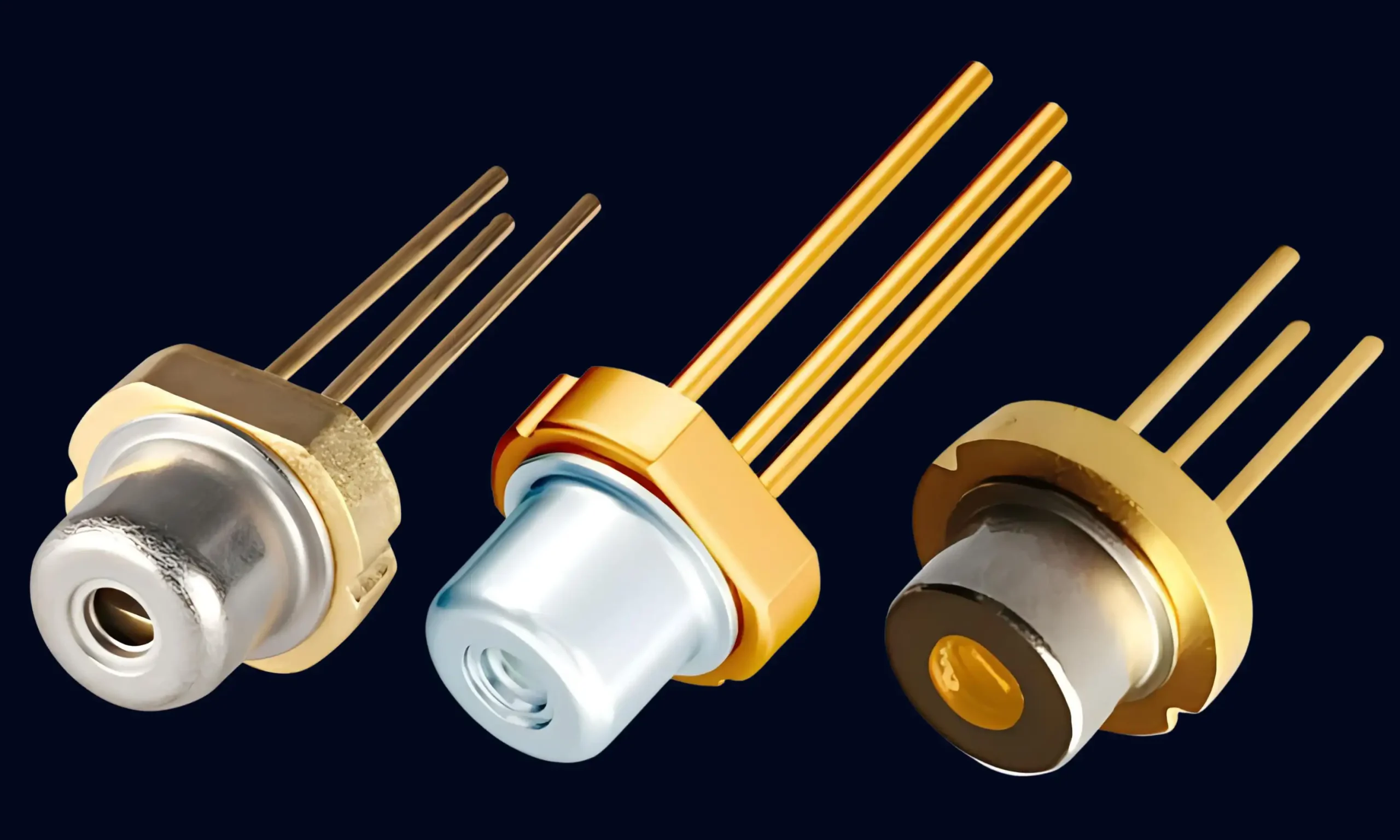
Additional Laser Diode Types
Beyond the standard edge-emitting and VCSEL types, some other exotic laser diode structures offer advantages for specialty applications. Let’s have a look at their characteristics.
Quantum Well Laser Diodes
- Made from layered structure of thin semiconductor films with alternating compositions and bandgaps.
- Confines carriers in the quantum well regions, enhancing laser efficiency.
- Requires lower threshold current density for lasing.
- Enables wavelength tuning by tailoring well and barrier dimensions.
- Produces light output at shorter wavelengths than conventional diode lasers.
- Widely commercialized laser type due to high efficiency and performance.
So, quantum well lasers provide major improvements in threshold, efficiency, and wavelength flexibility compared to traditional diode lasers.
Quantum Cascade Laser Diodes
- Emit in the mid-infrared region from 3-50 μm wavelength.
- Based on intersubband electronic transitions in a stacked heterostructure.
- Carriers tunnel through the cascade of quantum wells, emitting multiple photons per electron.
- Each active region is engineered for a specific transition to set the wavelength.
- It operates at room temperature, unlike other mid-IR lasers.
- Used in spectroscopy, gas sensing, and free space communications.
Quantum cascade lasers open the mid-infrared spectrum for applications like molecular fingerprinting and trace gas detection with their unique cascaded photon generation approach.
External Cavity Laser Diodes
- Use external reflective and dispersive optics to control properties.
- Includes lenses, diffraction grating, and volume Bragg grating.
- Optics provide wavelength filtering and optical feedback.
- Enables narrow linewidth tunable laser output.
- Single longitudinal mode is possible by suppressing side modes.
- Adjusting grating angle or temperature tunes wavelength.
- Used in spectroscopy, sensing, and medical applications.
So, external cavity diode lasers leverage external optics to control properties like wavelength, linewidth, and spatial mode compared to monolithic diode lasers. This enables tunable narrowband laser sources.
These exotic laser designs leverage quantum mechanical and bandstructure engineering techniques to optimize properties like efficiency, wavelength, and operating temperature beyond conventional diode lasers. Their emergence has expanded the utility of semiconductor lasers across the optical spectrum.
Summary
Laser diodes contain an active light-emitting semiconductor material like gallium arsenide within an optical cavity formed by cleaved facets or mirror layers. When injected with current, laser light is generated through spontaneous and stimulated emission, becoming rapidly amplified above the lasing threshold.
Important diode laser varieties for fiber optics include edge-emitting lasers like Fabry-Perot and DFB, surface-emitting VCSELs, quantum wells, quantum cascades, and external cavity lasers. Each offers particular advantages in terms of output power, efficiency, wavelength control, beam quality, and manufacturing.
Matching the laser diode design and specifications to the intended fiber optic system and network requirements is crucial for optimal performance. Laser diodes will continue serving as essential light sources powering high-speed fiber optic telecommunications.
FAQ
How do laser diodes work in optical fiber communication?
Laser diodes generate a highly focused and coherent light beam. In fiber optic communication, this light is injected into the optical fiber. As the light travels through the fiber, it experiences minimal attenuation due to the total internal reflection phenomenon. This allows for efficient transmission of information over long distances.
What are some limitations of using laser diodes in optical fiber communication?
➤ Cost: Manufacturing high-quality laser diodes can be expensive compared to some alternative light sources.
➤ Non-linear effects: At high transmission powers, non-linear effects within the fiber can distort the signal, requiring signal regeneration over long distances.
➤ Safety: Laser diodes emit concentrated light, requiring proper safety precautions to avoid eye damage during installation and maintenance.
What is the difference between a laser diode and a LED used in fiber optic communication?
Both laser diodes and LEDs emit light, but they differ significantly in their characteristics and suitability for fiber optic communication:
➤ Laser diode: Generates a highly focused, coherent light beam at a specific wavelength. This allows for efficient transmission over long distances with minimal signal loss.
➤ LED: Emits light with a broader spectrum and lower directionality. While LEDs can be used for short-distance communication, their limitations in power, wavelength range, and susceptibility to dispersion make them less suitable for high-speed and long-distance applications compared to laser diodes.
How do manufacturers ensure the safety of laser diodes used in fiber optic communication equipment?
Laser diodes are classified into different safety classes based on their potential for causing eye damage. Manufacturers implement several safety measures:
➤ Enclosed packaging: Laser diodes are housed within enclosures that prevent direct exposure of the emitted light.
➤ Warning labels: Equipment incorporating laser diodes is labeled with appropriate warnings and safety instructions.
➤ Interlocks and safeguards: Some systems may have interlocks that disable the laser if the housing is opened or safety protocols are not followed.
What are the different materials used to fabricate laser diodes for optical fiber communication?
The specific materials used for laser diodes depend on the desired operating wavelength:
➤ Gallium Arsenide (GaAs): Commonly used for short-wavelength (800-900 nm) laser diodes due to its efficient emission properties.
➤ Indium Phosphide (InP): Suitable for longer wavelength (1300-1600 nm) laser diodes, offering high performance and reliability in these communication bands.
➤ Quantum well structures: Advanced materials incorporating thin layers of different semiconductors can be used to achieve specific wavelengths and improve performance characteristics.